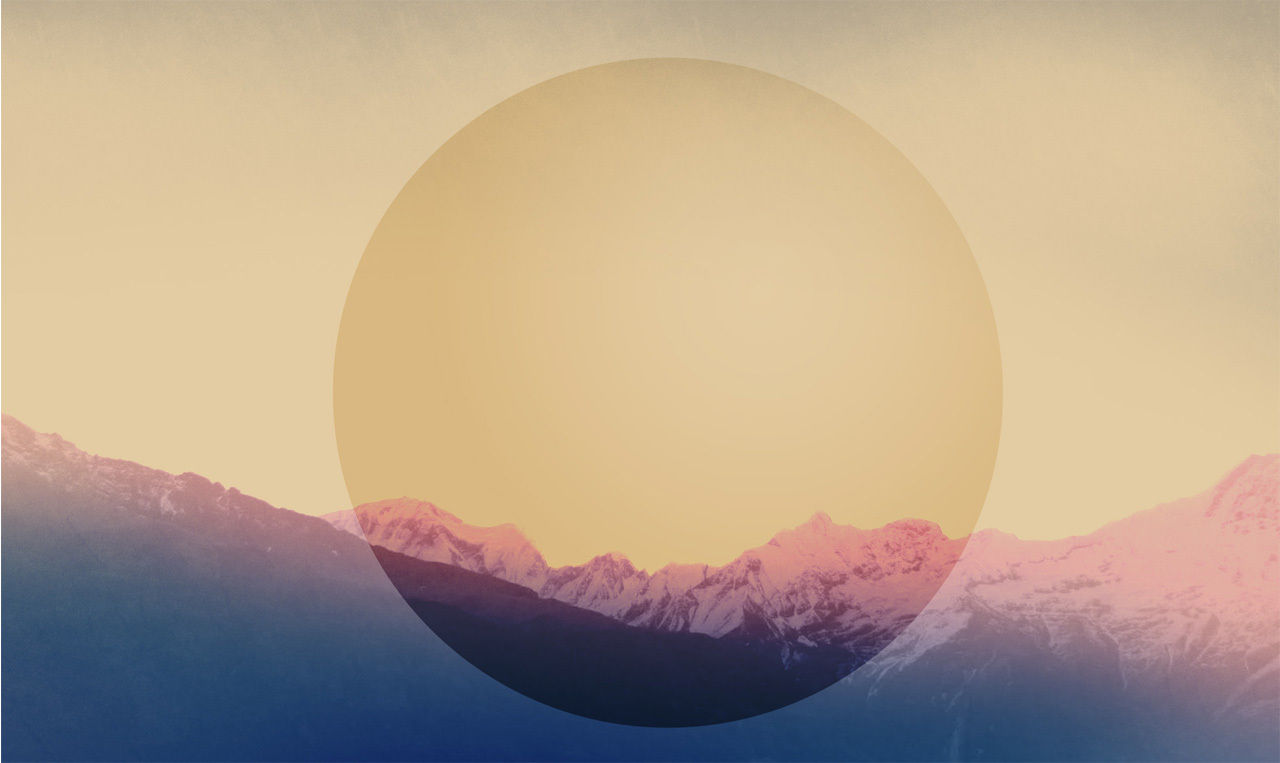
About My Projects
I am a research scientist in the field of medical imaging, working for KurtLab at the University of Washington. I recently earned my Ph.D. in Mechanical Engineering from The University of Washington in 2024. My current research is in developing MR-compatible devices and signal processing for diagnostic purposes and determining the mechanical properties of the human brain in-vivo. I have my M.Eng. in Mechanical Engineering, specializing in Medical Device Engineering, from Stevens Institute of Technology and my B.S. in Biomedical Engineering from The College of New Jersey. My undergraduate research was in assistive devices, with my independent research project being the development and testing of a five-fingered 3-D printed gip and pinch force augmenting exoskeleton for the human hand.
Main Project: Ultra High Field MR Elastography
Magnetic resonance elastography (MRE) is a technique for determining the mechanical response of tissue using both applied harmonic deformation of the tissue and magnetic resonance imaging (MRI). Performing MRE and gathering mechanical information on the brain can provide information on different structures within the tissue, for example, cell density, myelination, and inflammation, to potentially help diagnose diseases, for example, Alzheimer’s disease. Currently, studies investigating the diagnosis of these diseases using MRE are performed at 1.5T and 3T, which are the conventional magnetic field strengths of human MRI scanners. However, there have been a few attempts at performing MRE on the human brain at the ultra-high field strength 7T.
Sub-Project 1 | Design and Testing of MRE Actuators
In MRE, mechanical waves generated by the actuator, usually transmitted in a burst of specified duration, must be synchronized with the MR signal excitation. Additionally, this actuator must be used in an ultra-high MR setting, so the tissue-contacting portion of the actuator must contain only ultra-high MR-safe materials. Common electromagnetic and piezoelectric actuators are inherently made of metals and may not be ultra-high field MR-safe. With a pneumatic design, the active metallic components can be stored in the equipment room, while all of the tissue-contacting components used in the scanner room can therefore be made entirely of plastics. However, both acoustic wave attenuation and synchronization with the scanner can present an issue with this type of design due to the time delay of the acoustic waveform moving from the active pneumatic driver to the end-effector.
For this sub-project, I designed, tested, and validated a 7T compatible pneumatic MRE actuator. This actuator has been used for both phantom and human studies and is variable in amplitude and frequency. The frequency response and amplitude of the actuator at 50-100Hz has been quantified and the ergonomic pillow design for use in the brain has been optimized for both vibration transmission and subject comfort.
Journal Publication: [J4]
Conference Publications: [C18], [C20], and [C22-C24]

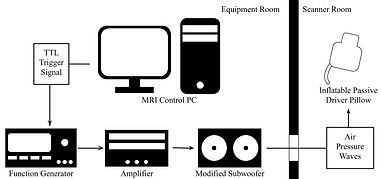
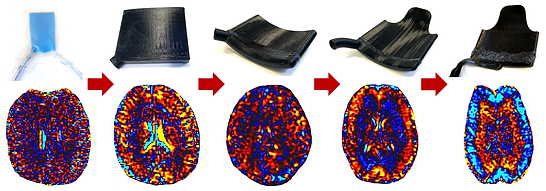
Sub-Project 2 | Noise Reduction in High Resolution MRE
When moving to 7T from 3T and therefore to higher resolutions, noise is inherently going to increase. It has been shown that quantitative values of MRE, i.e. the magnitude of the complex shear modulus estimate (|G*|), are sensitive to changes in SNR, so 7T MRE can present a challenge of not only quality but accuracy. While commonly-used filtering techniques (e.g., Gaussian, Median) can increase the SNR of MRE phase data (to combat low SNR in high-resolution scans), this also can blur fine physiological features, decrease the effective resolution, resulting in artificially increased |G*|. By utilizing a Marchenko-Pastur Principal Component Analysis (MP-PCA) denoising algorithm (developed for DTI image processing at NYU), and exploiting the intrinsic redundancies in MRE acquisition, physiological structures can be preserved, and high resolutions can be maintained. Our pilot study has shown an increase in octahedral shear strain based signal-to-noise ratio (OSS-SNR) after using MP-PCA denoising, and has demonstrated a relationship between resolution, OSS-SNR, and |G*| that requires further investigation.
At these high resolutions, we also see irregularities due to errors along phase wrapping lines when utilizing standard reconstruction methods during the scanner’s image reconstruction phase. These irregularities are not randomly distributed noise and therefore cannot be resolved using denoising. We are therefore investigating improvements in OSS-SNR of denoised images collected using standard reconstruction (sum-of-squares of 32 receive-coils) and images that have been reconstructed by utilizing the raw data from each of the 32 receive-coils and recombining it using an adaptive technique based on individual coil signal quality and magnetic field inhomogeneities. The irregularities in DICOM phase images, especially in the center of the brain, commonly arise due to the low SNR and following challenges for the coil combination algorithm. Also, the imperfection in phase wrapping can be clearly seen in DICOM phase difference images (in a shape of a ring). Such reconstruction errors propagate into the curl images and affect significantly the final quantitative values of shear stiffness. In this ongoing study, using the advanced Gadgetron image recognition, we have been able to avoid those errors, which is resulting in a more accurate stiffness estimation.
Conference Publications: [C14-C17]
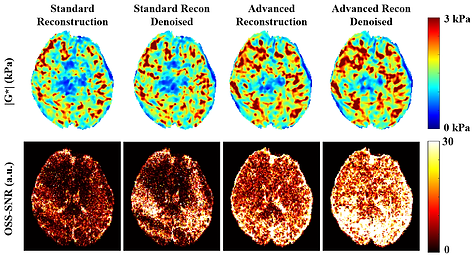
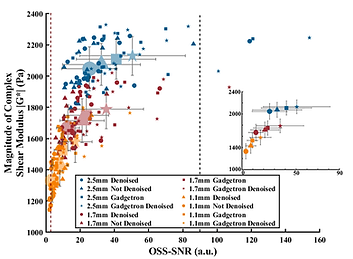
Sub-Project 3 | 7T vs 3T Comparison at Multiple Resolutions Using Nonlinear Inversion
Direct inversion methods require a variety of simplifying mechanical assumptions that, while result in fast processing, may contribute to the observed resolution-dependent |G*| results for brain tissue. Because these |G*| values are calculated directly using displacement maps, it is very likely that higher spatial wave sampling rate is a contributor to this resolution-dependent differences. Additionally, using direct inversion requires the application of many spatial and temporal smoothening filter (e.g., curl filtering and Romano filtering), reducing both the effective property map resolution (and therefore the benefit of moving to high resolutions), and the benefit of using non-smoothening denoising methods like the MP-PCA denoised applied in the previous Chapter. Therefore, it became clear that moving to a finite element, optimization based, nonlinear inversional algorithm was necessary for better understanding of the mechanical properties of highly inhomogeneous and complex tissues such as the brain, as well as for determining clinical applicability of high resolution MRE. Nonlinear viscoelastic inversion, as developed by McGarry, et al., has been extensively used and validated for MRE of the human brain at various resolutions at 3T (3.00 mm – 1.25 mm) and can calculate heterogeneous mechanical properties of tissue, reducing model-data mismatch that occurs in direct inversion methods. This will allows us to better model the small heterogeneous structures in the brain that are also visible in other imaging modalities.
Also, one of the main benefits of performing high resolution MRE at 7T is that other high resolution imaging modalities that are only available at 7T can also be captured for the same subjects in the same imaging sessions. The highest resolution that was performed in previous Chapters on the entire healthy young-adult cohort, 1.1 mm isotropic resolution, was specifically targeted because it is the resolution that is used during 7T Diffusion Tensor Imaging (DTI). By performing MRE at a matched resolution to these highly resolved 7T MRI sequences, we can determine the relationship between stiffness and these other properties like microstructural integrity, water diffusion, tissue anisotropy, perfusion, etc., as these contribute to the heterogeneity and anisotropic material properties of brain tissue. We established high resolution MRE at 7T with a custom 2D multi-slice single-shot spin-echo EPI sequence, using the Gadgetron advanced image reconstruction framework, applying Marchenko-Pastur Principal Component Analysis denoising, and using Nonlinear Viscoelastic Inversion. These techniques allowed us to accurately calculate the viscoelastic properties of the whole human brain at 1.1 mm isotropic imaging resolution with high OSS-SNR and accuracy. Using phantom models and 7T MRE data of eighteen healthy volunteers, we demonstrate the robustness of our method at high-resolution while quantifying the feasible tradeoff between resolution, OSS-SNR, and scan time. Using these post-processing techniques, we significantly increased OSS-SNR at 1.1 mm resolution with whole-brain coverage by approximately 4-fold and generated accurate elastograms with high anatomical detail. Performing high-resolution MRE at 7T on the human brain can provide information on different substructures within brain tissue based on their mechanical properties, which can then be used to diagnose pathologies (e.g., Alzheimer’s disease), indicate disease progression, or better investigate neurodegeneration effects or other relevant brain disorders, in vivo.
Journal Publication: [J1]
Conference Publications: [C4] and [C14]
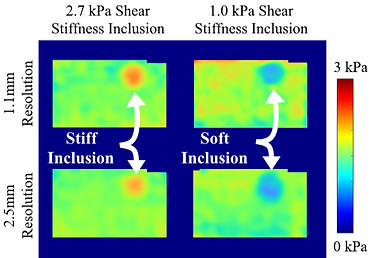

Sub-Project 4 | Multifrequency MRE at 7T
Most biological tissues, including the human brain, exhibit a frequency-dependent mechanical response which has yet to be explored at 7T. I am therefore investigating the frequency dependence of the mechanical properties of the brain at high resolution using 7T MRE, first validating this method using a linearly elastic phantom. In this ongoing study, I utilize the custom MRE actuator and three different versions of our high resolution MRE scanning protocol, scanning at 40Hz, 60Hz, and 80Hz. The results from our initial multifrequency pilot scan match the known frequency dependent (shear stiffening) behavior of the human brain. In the future, this multifrequency MRE protocol can be used to determine region specific frequency-dependent mechanical properties as it has in the past at 3T, but at the ultra-high resolution possible at 7T. By obtaining the multifrequency response of the human brain at the ultra-high resolution possible using 7T MRE, we can develop subfield specific material models. These material models can therefore be used to inform FE brain models and therefore simulate brain motion at the high frequency impacts accurately. Understanding brain movement and tissue deformation mechanisms during these impacts is a powerful strategy for predicting brain injury and developing preventative equipment.
Conference Publication: [C8] and [C16]

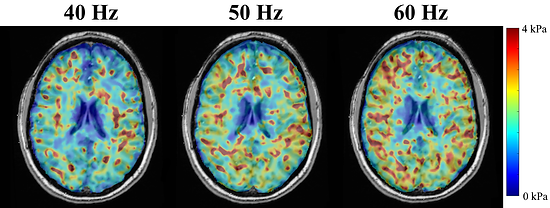
Sub-Project 5 | MRE as a Potential Biomarker for Alzheimer’s Disease
Alzheimer’s disease (AD) is the leading cause of dementia in the aging population worldwide, and a progressive neurodegenerative condition of enormous socio-economic impact. The main pathogenic hallmarks of the disease are the progressive extracellular accumulation of β-amyloid (Aβ) plaques and intraneuronal aggregation of neurofibrillary tau tangles. Identification of non-invasive correlates of these pathologies to aid diagnosis in the earliest stages of disease will help to afford improved disease management and prevention. One such non-invasive correlate that has received growing attention involves the study of the mechanical properties of the brain via MRE, as it is becoming increasingly clear that tissue mechanics are related to the underlying cellular microstructure and overall health state of an organ. Pivotal studies applying MRE at 3T have shown a progressive softening of white and gray matter tissue in AD patients compared to healthy controls (especially in the frontal, parietal and temporal lobes) in line with the known topography of AD pathology. One of the limitations of this approach, however, is that the underlying microstructural causes of tissue viscoelasticity variations cannot be directly determined, hence there is a lack of physical understanding regarding the meaning of these parameters in the context of dementia and neurodegeneration. This difficulty limits the current predictive value of MRE for early diagnosis and for the assessment of individual risk of developing cognitive decline. In this final aim, we will test the efficacy of the MRE methods developed in the previous Aims on extracting MRE biomarkers in patient with AD and Mild Cognitive Impairment (MCI). For the first part of this Aim, we will develop a framework for correlating regional 7T MRE metrics and amyloid PET metrics, and perform this regional correlation on a small cohort of older healthy adults. In the second part of this Aim, we will perform high resolution 7T MRE and 7T DTI on a cohort of AD or MCI patients and a cohort of age-matched healthy controls who have all undergone amyloid PET scans and cognitive testing in a previous study. We will then utilize our previously validated advanced frameworks and post-processing techniques and correlate the regional complex shear modulus from MRE, fractional anisotropy and diffusivity from DTI, the previously collected beta-amyloid PET, and cognitive testing results to determine microstructural changes as a result of neurodegeneration.
In this ongoing project, we are working to bring forward a novel framework by combining MRE with specialized, ultrahigh resolution, MR neuroimaging at 7T, wherein this multi-modal approach will enable the joint analysis of biomechanical, connectomic and pathologic markers in AD patients and bring novel insights in our understanding of the mechanisms of AD onset and progression.
Conference Publications: [C1-C3], [C6], [C9], [C11], and [C13]



Graduate Side Projects and Undergraduate Research
Project |01
Graduate Research Project | BioInterface Characterization through MRE
Most tissues consist of multiple layers and membranes, and abnormalities such as tumors, all of which interact through bio-interfaces. Detailed mechanical characterization of bio-interfaces under physiological conditions (ideally in vivo) is essential for accurate diagnosis, prognosis, and effective treatment on biomedical applications. With the help of new MR imaging modalities such as MR elastography (MRE) and amplified MRI (aMRI), now we are capable of investigating the short term temporal variation of strain fields in vivo in the vicinity of bio-interfaces.
Journal Publication: [J4]
Conference Publications: [C7] and [C8]
Project |02
Graduate Research Project | Nonlinear Modal Analysis in the Human Brain
We aim to investigate the fundamental nonlinear dynamics of a simplified finite element (FE) brain model. We modeled the brain as a long cylinder with dimensions similar to that of the human brain with a thin membrane embedded in the center. The cylinder is subjected to sudden harmonic rotations applied at its external boundary around the main vertical axis. We will also test this in a similar MRI phantom setup. This 3D printed setup will provide a high deceleration to a brain-mimicking silicone phantom with a thin plastic membrane to represent the falx cerebri.
Conference Publication: [C6]
Project |03
Undergraduate Research Project | Five-Fingered Powered Exoskeleton for the Human Hand
Over 795,000 Americans suffer from disorders, such as arthritis and stroke, or injuries to the hand that results in decreased grip strength and/or the ability to hold objects. The main objective of this research is to design and produce a wearable device to augment the pinching and grasping efforts of the wearer. I improved the design of an orthotic five-fingered powered exoskeleton for the human hand previously developed in this laboratory. A new control system was integrated into the device in order to improve the functioning of battery-powered electric motors that can independently augment the movement of each of the five fingers. After the basic performance of the device is verified experimentally, human testing of the device will commence. The electrical activity of muscles in the forearm will be recorded to provide a quantitative measure of the effectiveness of the device to reduce the hand muscle activation necessary to produce grasping and pinching efforts. We hypothesize that while using the orthotic device, as compared to barehanded efforts, subjects will use significantly decreased muscle efforts during pinching and grasping movements.
Journal Publication: [J3]
Conference Publication: [C9]